Stressing the metabolic powers of fibroblast growth factor 21
The global prevalence of obesity has continued to rapidly increase over the last few decades. As well as the range of metabolic disorders associated with obesity, diseases like diabetes, which was the second leading cause of death related to obesity in 2015, all add to the global burden of disease (1). Currently, the options for treatment of obesity and its associated disorders are limited. Soluble pro-inflammatory cytokines including interleukin-6 and granulocyte macrophage colony stimulating factor have exhibited profound beneficial metabolic effects. These include promotion of fat oxidation and insulin sensitivity and reduction in food intake and body weight, respectively (2,3). The adipokines adiponectin and leptin may also have a positive effect on body weight regulation (4,5). Additionally, overexpression of interleukin-10 protected mice from diet induced inflammation and insulin resistance in skeletal muscle (6). Also emerging as an appropriate therapeutic agent is the soluble fibroblast growth factor 21 (FGF21) (Figure 1) (16). Early investigations in which FGF21 was administered to mouse models of obesity and diabetes revealed that it reduced plasma glucose levels, lowered mean body weight and reversed hepatic steatosis (7-9). In monkeys, it has been shown that FGF21 decreases sweet preference via dopamine signalling (12). Soberg et al. highlighted that in humans, the preferences for sweet tasting substances is determined by genetic variation of the fgf21 gene (15). While native FGF21 has an unfavorable pharmacokinetic profile, a number of pharmaceutical companies have developed FGF21 analogues that appear more suitable for use in humans (16). Published data from clinical trials administering two FGF21 mimetics, LY2405319 and PF-05231023, to patients with type 2 diabetes have demonstrated that this approach is safe (13,14). LY2405319 was found to significantly reduce body weight and fasting insulin levels of patients receiving the treatment compared to placebo after 28 days but only showed a dose dependent trend of lowering fasting glucose levels (14). After 25 days of treatment with PF-05231023, patients had a significant reduction in body weight compared to the placebo group. Patients receiving PF-05231023 also showed a trend towards lower glucose and insulin levels compared to subjects receiving placebo although they were not statistically different (13). Interestingly, in a larger clinical trial of PF-05231023 involving obese subjects with or without type 2 diabetes, no significant reduction in body weight could be observed (17). As clinical trials using these and other newly developed FGF21 mimetics continue, an alternative therapeutic approach aimed at augmenting endogenous FGF21 levels and/or activity is being pursued (16). Many studies have demonstrated that FGF21 expression can be upregulated in multiple tissues in response to stress (18). Sources of stress, including amino acid deprivation and endoplasmic reticulum stress, can initiate the integrated stress response (ISR) pathway by activating one of four kinases, PKR-like ER kinase (PERK), double-stranded RNA-dependent protein kinase (PKR), heme-regulated eIF2α kinase (HRI) or general control non-depressible 2 (GCN2) (Figure 2). These kinases can then phosphorylate eIF2α, a central component of ISR signalling, which leads to upregulated activity of the transcription factor activating transcription factor 4 (ATF4) and subsequent increased transcription of the Fgf21 gene (18).
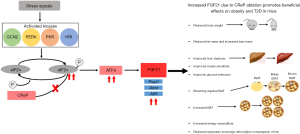
In a recent publication in Hepatology, Xu and colleagues generated a mouse model, CRePLKO, in which constitutive repressor of eIF2α phosphorylation (CReP) was specifically knocked out in the liver (19). CReP is a subunit of the eIF2α phosphatase complex that is involved in the downregulation of eIF2α phosphorylation. As expected, a hepatic knockout of CReP resulted in increased levels of phosphorylated eIF2α in the liver of CRePLKO mice compared to wild-type (WT) (Figure 2). The CRePLKO mice were grossly normal in appearance with minimal impact on the protein synthetic and secretory function of the liver observed. Their liver to body weight ratio was 14% lower than WT. The CRePLKO mice contained strikingly brown-coloured subcutaneous inguinal white adipose tissue (iWAT). Prior to being placed on a high-fat diet (HFD), the CRePLKO mice had 17% lower body weight compared to WT, a difference that grew to 32% after both groups of mice were placed on an HFD for 12 weeks. After 12 weeks on an HFD, the CRePLKO mice had a significantly lower fat mass percentage than the WT, paralleled by a higher lean mass percentage, which suggests a reduction of adiposity. In comparison to the WT mice, the CRePLKO mice exhibited a higher energy expenditure but lower respiratory exchange ratio both before and after being on an HFD. This is indicative of a higher consumption of fat as an energy source in the CRePLKO mice consistent with the brown-coloured iWAT found in them. Insulin sensitivity and glucose homeostasis was improved in the CRePLKO mice both before and after being on an HFD. This allowed the mice to maintain normal glucose and serum insulin levels while on an HFD for 12 weeks. The observed increases in energy expenditure and insulin sensitivity with elevated FGF21 levels are consistent with those of previous studies (10,11). The livers of the CRePLKO mice were in a noticeably healthier condition than the WT mice, which developed clear signs of hepatic steatosis, after 12 weeks on an HFD (Figure 2).
These phenotypical differences between the CRePLKO and WT mice were underpinned by key differences at the molecular level between the two types of mice. With the higher level of eIF2α phosphorylation in the livers of CRePLKO mice, there was also an elevated level of the downstream ISR signalling proteins, ATF4 and ATF5. The team also detected higher mRNA levels of genes including Atf5, Asns, and Psat1, which are regulated by the transcription factors ATF4 and ATF5. The aforementioned browning of iWAT in CRePLKO mice was accompanied expectantly with increased expression of brown adipocyte markers Ucp1, Dio2 and Elovl3 in WAT and brown adipose tissue (BAT). Without the degree of hepatic steatosis observed in the WT mice, there were lower levels of proteolytic activation of SREBP-1c and expression of its target lipogenic genes in the livers of CRePLKO mice as expected. The greater insulin sensitivity in the CRePLKO mice corresponded with higher insulin-mediated AKT phosphorylation in their livers and WAT compared to WT. Of most interest were the higher levels of FGF21 mRNA in the liver and FGF21 protein in the serum of CRePLKO mice, which was maintained throughout HFD feeding. These levels were in fact similar to the levels seen in fasted WT mice. When CRePLKO mice were fasted, the FGF21 levels increased further from their already elevated level.
To further elaborate on their findings, Xu et al. used two further mouse models, protein phosphatase 1 regulatory subunit 15bloxP (Ppp1r15bloxP) and double knockout (DKO) mice. By injecting an AAV-Cre vector into Ppp1r15bloxP mice, Xu et al. were able to ablate CReP expression in the liver of adult mice. Following the injection of AAV-Cre, the adult Ppp1r15bloxP mice exhibited many features observed in the CRePLKO mice including, upregulation of Fgf21, Asns and Psat1 in the liver, no elevation of ER stress markers and increased expression of Ucp1 and Elov3 in WAT and BAT. Body weight and blood glucose levels in Ppp1r15bloxP mice were reduced by 6% and 20%, respectively, within three days of the AAV-Cre injection. Ppp1r15bloxP mice that had been on an HFD also saw a reduction in their body weight and blood glucose levels by 20% and 45%, respectively, two weeks after being injected with AAV-Cre. Improvements in insulin sensitivity and liver steatosis, as well as a decrease in fat mass were also observed in Ppp1r15bloxP mice on an HFD following the AAV-Cre injection. The results observed in the AAV-Cre injected Ppp1r15bloxP mice suggest that activation of ISR in the liver is a viable target for the treatment of obesity and is capable of providing multiple beneficial metabolic effects.
The DKO mouse was generated by crossing CRePLKO mice with Fgf21loxP mice, which do not express FGF21. All the beneficial effects of CReP ablation seen in CRePLKO mice were negated by the loss of FGF21 in the DKO mice, which were more similar to WT mice. There was no browning of WAT seen in DKO mice with Ucp1 and Elovl3 mRNA levels similar to those in WT mice. The energy expenditure, respiratory exchange ratio, insulin sensitivity and glucose homeostasis in the DKO mice were also all similar to WT mice. The generation of the DKO mice by Xu et al. eloquently demonstrated that the all the benefits seen in the CRePLKO mice were dependent on presence of FGF21.
The protective benefits of ISR signalling and the central role that eIF2α plays clearly shown by Xu et al. mirrors the findings of Scheuner and colleagues who studied a mouse model in which eIF2α could not be phosphorylated (20,21). Eif2s1tm1Rjk mice were generated by using gene targeting to mutate the Ser51 phosphorylation site of eIF2α to prevent its phosphorylation (20). Homozygous Eif2s1tm1Rjk mice died within 18 hours of birth due to hypoglycemia and were found to have only 20% of the serum insulin level of WT mice (20). Heterozygous Eif2s1+/tm1Rjk mice on an HFD gained significantly more body weight, had reduced energy expenditure and a higher respiratory exchange ratio in comparison to WT mice on the same diet (21). After 5 weeks on an HFD, heterozygous Eif2s1+/tm1Rjk mice developed severe glucose intolerance compared to WT mice on an HFD in which only a minor change in glucose intolerance was observed in the same timeframe (21). The work of Xu et al. and Scheuner et al. highlight the importance of the ISR pathway and provide a possible therapeutic target in eIF2α to protect against the metabolic impact of obesity via the upregulation of endogenous FGF21. Drugs that inhibit eIF2α phosphatases like CReP include salubrinal, sephrin1, guanabenz and nelfinavir. These drugs are available but it is important to note that targeting the ISR pathway does not have uniform consequences in all cell types and may result in unwanted side effects (22).
In addition to the ISR pathway there are alternative pathways that have been demonstrated to be involved in the upregulation of endogenous FGF21 expression. Metformin, for example, was found to increase the level of FGF21 expression in obese mice (23). Increased liver expression of FGF21 was observed in Sprague-Dawley rats that had received a combination of docosahexaenoic acid and triiodothyronine (24). Our own work has highlighted that when haematopoietic cells express TNFSFS14, there is a concomitant increase in hepatic FGF21 levels (25). More importantly, the increased FGF21 expression aligned with an improved metabolic profile (25).
Gene therapy is also a novel avenue being pursued to elevate the endogenous level of FGF21 to treat obesity. Jimenez and colleagues used a variety of adeno-associated virus vectors to overexpress FGF21 in the liver of C57BL/6 mice on an HFD, the liver or epididymal WAT of ob/ob mice (a model of obesity) and skeletal muscle of WT mice (26). Gene therapy induced FGF21 overproduction in each mouse model and irrespective of the tissue source of increased FGF21, FGF21 prevented body weight gains that were observed in control mice treated with null vectors (26).
Overall, there have been exciting developments in the search for viable therapeutic options for the treatment of obesity and the metabolic disorders related to it. The work of Xu et al. clearly demonstrates the protective benefits of engaging the ISR pathway against the metabolic dysfunction of obesity. It represents another piece of evidence that highlights the beneficial role that FGF21 can play in the treatment of metabolic diseases. However, optimism about a treatment must be tempered with caution as there still remains much to be elucidated about how these pathways interact within the body and what impact long-term supra-physiological levels of FGF21 may have on the body.
Acknowledgements
Funding: We kindly acknowledge funding from the Royal Perth Hospital Medical Research Foundation to MPS and VBM.
Footnote
Provenance and Peer Review: This article was commissioned by the editorial office, AME Medical Journal. The article did not undergo external peer review.
Conflicts of Interest: The authors have completed the ICMJE uniform disclosure form (available at http://dx.doi.org/10.21037/amj.2018.09.05). The authors have no conflicts of interest to declare.
Ethical Statement: The authors are accountable for all aspects of the work in ensuring that questions related to the accuracy or integrity of any part of the work are appropriately investigated and resolved.
Open Access Statement: This is an Open Access article distributed in accordance with the Creative Commons Attribution-NonCommercial-NoDerivs 4.0 International License (CC BY-NC-ND 4.0), which permits the non-commercial replication and distribution of the article with the strict proviso that no changes or edits are made and the original work is properly cited (including links to both the formal publication through the relevant DOI and the license). See: https://creativecommons.org/licenses/by-nc-nd/4.0/.
References
- Afshin A, Forouzanfar MH, Reitsma MB, et al. Health Effects of Overweight and Obesity in 195 Countries over 25 Years. N Engl J Med 2017;377:13-27. [Crossref] [PubMed]
- Carey AL, Steinberg GR, Macaulay SL, et al. Interleukin-6 increases insulin-stimulated glucose disposal in humans and glucose uptake and fatty acid oxidation in vitro via AMP-activated protein kinase. Diabetes 2006;55:2688-97. [Crossref] [PubMed]
- Reed JA, Clegg DJ, Smith KB, et al. GM-CSF action in the CNS decreases food intake and body weight. J Clin Invest 2005;115:3035-44. [Crossref] [PubMed]
- Fruebis J, Tsao TS, Javorschi S, et al. Proteolytic cleavage product of 30-kDa adipocyte complement-related protein increases fatty acid oxidation in muscle and causes weight loss in mice. Proc Natl Acad Sci U S A 2001;98:2005-10. [Crossref] [PubMed]
- Pelleymounter MA, Cullen MJ, Baker MB, et al. Effects of the obese gene product on body weight regulation in ob/ob mice. Science 1995;269:540-3. [Crossref] [PubMed]
- Hong EG, Ko HJ, Cho YR, et al. Interleukin-10 prevents diet-induced insulin resistance by attenuating macrophage and cytokine response in skeletal muscle. Diabetes 2009;58:2525-35. [Crossref] [PubMed]
- Coskun T, Bina HA, Schneider MA, et al. Fibroblast growth factor 21 corrects obesity in mice. Endocrinology 2008;149:6018-27. [Crossref] [PubMed]
- Kharitonenkov A, Shiyanova TL, Koester A, et al. FGF-21 as a novel metabolic regulator. J Clin Invest 2005;115:1627-35. [Crossref] [PubMed]
- Xu J, Lloyd DJ, Hale C, et al. Fibroblast growth factor 21 reverses hepatic steatosis, increases energy expenditure, and improves insulin sensitivity in diet-induced obese mice. Diabetes 2009;58:250-9. [Crossref] [PubMed]
- Li H, Wu G, Fang Q, et al. Fibroblast growth factor 21 increases insulin sensitivity through specific expansion of subcutaneous fat. Nat Commun 2018;9:272. [Crossref] [PubMed]
- Owen BM, Ding X, Morgan DA, et al. FGF21 acts centrally to induce sympathetic nerve activity, energy expenditure, and weight loss. Cell Metab 2014;20:670-7. [Crossref] [PubMed]
- Talukdar S, Owen BM, Song P, et al. FGF21 Regulates Sweet and Alcohol Preference. Cell Metab 2016;23:344-9. [Crossref] [PubMed]
- Talukdar S, Zhou Y, Li D, et al. A Long-Acting FGF21 Molecule, PF-05231023, Decreases Body Weight and Improves Lipid Profile in Non-human Primates and Type 2 Diabetic Subjects. Cell Metab 2016;23:427-40. [Crossref] [PubMed]
- Gaich G, Chien JY, Fu H, et al. The effects of LY2405319, an FGF21 analog, in obese human subjects with type 2 diabetes. Cell Metab 2013;18:333-40. [Crossref] [PubMed]
- Søberg S, Sandholt CH, Jespersen NZ, et al. FGF21 Is a Sugar-Induced Hormone Associated with Sweet Intake and Preference in Humans. Cell Metab 2017;25:1045-53.e6. [Crossref] [PubMed]
- Sonoda J, Chen MZ, Baruch A. FGF21-receptor agonists: an emerging therapeutic class for obesity-related diseases. Horm Mol Biol Clin Investig 2017;30. [PubMed]
- Kim AM, Somayaji VR, Dong JQ, et al. Once-weekly administration of a long-acting fibroblast growth factor 21 analogue modulates lipids, bone turnover markers, blood pressure and body weight differently in obese people with hypertriglyceridaemia and in non-human primates. Diabetes Obes Metab 2017;19:1762-72. [Crossref] [PubMed]
- Salminen A, Kaarniranta K, Kauppinen A. Integrated stress response stimulates FGF21 expression: Systemic enhancer of longevity. Cell Signal 2017;40:10-21. [Crossref] [PubMed]
- Xu X, Krumm C, So JS, et al. Preemptive activation of the integrated stress response protects mice from diet-induced obesity and insulin resistance via fibroblast growth factor 21 induction. Hepatology 2018; [Epub ahead of print]. [Crossref] [PubMed]
- Scheuner D, Song B, McEwen E, et al. Translational control is required for the unfolded protein response and in vivo glucose homeostasis. Mol Cell 2001;7:1165-76. [Crossref] [PubMed]
- Scheuner D, Vander Mierde D, Song B, et al. Control of mRNA translation preserves endoplasmic reticulum function in beta cells and maintains glucose homeostasis. Nat Med 2005;11:757-64. [Crossref] [PubMed]
- Pakos-Zebrucka K, Koryga I, Mnich K, et al. The integrated stress response. EMBO Rep 2016;17:1374-95. [Crossref] [PubMed]
- Kim EK, Lee SH, Lee SY, et al. Metformin ameliorates experimental-obesity-associated autoimmune arthritis by inducing FGF21 expression and brown adipocyte differentiation. Exp Mol Med 2018;50:e432 [Crossref] [PubMed]
- Vargas R, Riquelme B, Fernandez J, et al. A combined docosahexaenoic acid-thyroid hormone protocol upregulates rat liver beta-Klotho expression and downstream components of FGF21 signaling as a potential novel approach to metabolic stress conditions. Food Funct 2017;8:3980-8. [Crossref] [PubMed]
- Saunders BM, Rudnicka C, Filipovska A, et al. Shining LIGHT on the metabolic role of the cytokine TNFSF14 and the implications on hepatic IL-6 production. Immunol Cell Biol 2018;96:41-53. [Crossref] [PubMed]
- Jimenez V, Jambrina C, Casana E, et al. FGF21 gene therapy as treatment for obesity and insulin resistance. EMBO Mol Med 2018;10. [PubMed]
Cite this article as: Magno AL, Herat LY, Carnagarin R, Schlaich MP, Matthews VB. Stressing the metabolic powers of fibroblast growth factor 21. AME Med J 2018;3:97.