Review of patterns of lymphatic drainage in early-stage non-small cell lung cancer
Introduction
The presence of metastatic nodal disease has been shown to be the most important predictor of long-term disease-specific survival after surgical resection for non-small cell lung cancer (NSCLC) (1). Five-year overall survival is 65.7% for pathologic node negative “N0” patients and only 36.4% for patients with mediastinal “N2” disease (2).
To date, complete dissection with mediastinal stations has been proposed as the most accurate way of fully staging a patient with lung cancer, especially NSCLC (3). However, given the lack of a standardized recommendation for adequate lymphadenectomy and variable surgeon practices, only 50–60% of patients ultimately undergo complete dissection (4,5). Additionally, up to 40% of “node negative” patients who undergo complete resection relapse within 24 months after surgery, suggesting initial understaging of disease (1). Disease recurrence among patients with early stage disease underscores the need to improve surgical and histologic nodal staging to identify patients who may benefit from adjuvant treatments.
One of the keys to improving nodal staging is to advance our understanding of the lymphatic drainage in the thoracic cavity. This would also help in our understanding of the tumor-immune microenvironment, which is believed to play a role in the prognosis of early stage lung cancer (6). Tumor-draining lymph nodes are the sites at which antitumor immune responses are initiated (7) and are the preferential site of initial tumor metastases (8). This article will review the anatomy of the pulmonary lymphatic system, patterns of nodal metastasis in NSCLC, and opportunities for improvement in the clinical and pathologic detection of lymphatic spread of lung cancer.
Methods
This narrative review was conducted via a literature search in the PubMed database utilizing the following keywords: lung cancer, lymphatic, drainage. Both original research and review articles were considered for inclusion in this paper.
Anatomic patterns of pulmonary lymphatic drainage
The pulmonary lymphatic system is composed of vessels with a single lymphatic endothelial cell wall with a discontinuous basement membrane. Lymphatic channels transport antigens and antigen-presenting cells from peripheral lung tissue to lymph nodes and aid in the clearance of interstitial fluid. Within lung tissue, lymphatic channels frequently course in parallel to major airways and bronchioles as well as near intralobular arterioles and veins. Only about 3.6–19% of alveoli within the lung parenchyma are associated with lymphatic structures (9). The lung surface is additionally drained by a subpleural lymphatic network.
The lymphatic system of the lungs can be divided into the superficial (or pleural) and deep systems (10), with the former representing the main drainage system for the pulmonary segments. These two draining systems frequently intersect before terminating in tracheobronchial or hilar nodes. These nodes are clinically designated as level N1, or level 10, lymph nodes and intraparenchymal lymphatic drainage to these hilar nodes are typically thought to result in subsequent drainage to mediastinal, or N2, lymph nodes (11).
However, direct lymphatic drainage from intraparenchymal lymphatics to mediastinal lymph nodes has also been described. Historical descriptions of so-called “skip” lymphatic drainage to N2 nodes was published as early as 1932 by Rouvière et al. (12) and subsequently demonstrated in cadaveric studies by Riquet et al. (13). Direct drainage from peripheral lung tissue to the mediastinal nodes was seen after dye injection in almost 25% of lymphatic vessels. Despite the limitations of cadaveric lymphatic mapping studies, an approximately 20–38% rate of “skip” metastases in lung cancer is observed in current literature (11).
More recent cadaveric studies (13,14), as summarized in Table 1, have supported these findings of routes that directly drain to the mediastinum, providing a possible explanation for the clinical observation of “skip” metastases to N2 level nodes seen in lung cancer. Multiple studies have demonstrated that the highest percentage of direct mediastinal drainage is seen in the right lung, with over half of these direct drainage pathways observed in the right upper lobe (10,14).
Table 1
Trial | Year | Tracer | Method of injection | # of patients | Patient population | SLN ID rate | Accuracy |
---|---|---|---|---|---|---|---|
Isosulfan blue dye | |||||||
Little et al. (15) | 1999 | Isosulfan blue | Transpleural | 36 | NSCLC | 47% | – |
Radiocolloid | |||||||
Liptay et al. (16) | 2000 | Tc-99 sulfur colloid | Transpleural | 52 | Suspected NSCLC | 82% | 94% |
Rzyman et al. (17) | 2006 | Tc (albumin, sulfur, Nanocis, and tin) colloid | Transpleural | 110 | Clinical N0 NSCLC | 100% | 95% |
Liptay et al. (18) | 2009 | Tc-99 sulfur colloid | Transpleural | 46 | Stage I NSCLC | 61.5% | 83.3% |
Nomori et al. (19) | 2009 | Tc-99 tin colloid | Transthoracic | 104 | Stage I NSCLC | 81% | – |
NIR | |||||||
Yamashita et al. (20) | 2012 | ICG | Transpleural | 61 | Clinical N0 disease | 80.3% | 78.7% |
Moroga et al. (21) | 2012 | ICG | Transpleural | 20 | Stage IA NSCLC | 80% | – |
Gilmore et al. (22) | 2013 | ICG | Transpleural | 38 | Suspected stage I/II NSCLC | 100%** | 100%** |
Hachey et al. (23) | 2017 | ICG | Transbronchial | 12 | Stage I NSCLC | 80% | – |
**, at optimized dose. SNL, sentinel lymph node; ICG, indocyanine green; NSCLC, non-small cell lung cancer; NIR, near infrared imaging.
Ndiaye et al. also demonstrated pathways of drainage into the thoracic duct from the apical segment of the right upper lobe (14). In these studies, the right middle lobe also has pathways draining directly to the mediastinum in 18.6% of injected pathways (13) to 37.5% (14) of injected segments.
The visceral or subpleural lymphatic pathway, which is associated with draining lymph from the surface of lung parenchyma, was first studied more closely by Fourdrain et al. (24). This study, while small (n=20), provided evidence that lymphatic drainage of the peripheral lower lobes could follow an intersegmental pathway, possibly affected by the anatomy of each individual’s fissure.
In addition to the pulmonary lymphatic anatomy described above, additional de novo lymphangiogenesis, or new vessel formation in the lungs, is observed in patients with chronic obstructive pulmonary disease (COPD), present in 40–70% of patients with a lung cancer diagnosis (25,26). Histologic analysis of tissue biopsies in patients with advanced COPD demonstrates a 133% increase in the number of alveolar parenchymal lymphatics compared to tissues of never-smoking patients without COPD. Lymphangiogenesis in COPD is associated with upregulation of chemokine ligand CCL21, which binds to activated dendritic cells within the lymphatics, and chemokine receptor D6, which degrades inflammatory chemokines and clears the endothelial walls of lymphatic vessels. Expression of CCL21 and D6 within lymphatic vessels is thought to facilitate the migration of CCR7-expressing immune cells to lymph nodes for antigen-presentation (27). The lymphatic remodeling seen in chronic lung disease may set the stage for lymphatic metastases, as NSCLC is known to express CCR7 and VEGF, triggering further lymphangiogenesis in the tumor microenvironment.
Patterns of nodal metastasis in NSCLC
The accuracy of preoperative lung cancer staging with PET/CT and mediastinal staging with mediastinoscopy and endobronchial ultrasound continues to improve and lend insight into patterns of nodal spread in NSCLC. However, rates of upstaging after lobectomy for clinical stage I lung cancer range from 8.5–27.5% and efforts to optimize nodal staging at the time of definitive surgery remain critical to identifying patients with missed metastatic disease (2,28,29).
A 2017 study by Lilo et al. (30) compared detection of nodal disease by PET/CT and endobronchial ultrasound-guided transbronchial needle aspiration (EBUS-TBNA) and highlighted the continued importance of obtaining histology to confirm mediastinal disease. While only 41.9% of PET positive lymph nodes were malignant, EBUS-TBNA identified an additional 7.4% malignant lymph nodes among PET-negative nodes. The sensitivity, specificity, and positive and negative predictive values of EBUS-TBNA cytology reported in this study were 90%, 100%, 100% and 88.9%. Additional real-world evaluations of EBUS-TBNA reveal that 33.3% of false negative EBUS cases with NSCLC could be attributed to non-sampling of EBUS-accessible lymph nodes that were later obtained at the time of surgery (31). A number of these pathologic positive nodes, which included both hilar and mediastinal nodal stations, were not PET-avid on preoperative imaging. The study findings underscore the need for a more systematic protocol for EBUS-TBNA lymph node sampling.
The direct pathways of lymphatic drainage previously mentioned could be associated with the prevalence of skip metastases. Studies which have systematically sampled nodes have demonstrated the presence of tumor in N2 nodal stations without affecting the N1 nodes. In Bille et al. (2), patients with clinically node negative early stage NSCLC underwent anatomic resection and lymphadenectomy with resection of at least 2 mediastinal stations, always including level 7. For right-sided tumors, sampling included lymph node stations 2 or 4R and/or 8 or 9. For left-sided tumors, sampling included stations 5 or 6 and/or stations 8 or 9. Among these early stage cancers, the incidence of N1 and N2 disease were 8 and 9 percent, with “skip” metastases (N2 lymph node involvement without N1 involvement) identified in 34% of patients. In addition, 16% of pathologic N2 patients in the study had mediastinal nodal metastases beyond lobe-specific drainage patterns. Overall, studies such as this have supported systemic lymph node sampling even in stage I NSCLC given the prevalence of these “skip metastases.”
In contrast, in a cohort of 1,047 patients, the ACOSOG Z0040 trial demonstrated that in 22.4% of patients who were previously deemed N0, occult micrometastases were found on further immunohistochemical analysis. This pattern was also associated with a worse prognosis and overall survival. In addition, this study also found no difference in long-term survival between patients with early stage NSCLC (T1 or T2, N0 or N1 non-hilar) who underwent mediastinal lymph node dissection versus sampling (4). More recent studies (32,33) have demonstrated similar results, with improved disease-free survival with radical lymph node dissection, though no improvement in overall survival. In the setting of this shift in recommendations from complete lymph node dissection (3) and towards a more targeted surgical staging for NSCLC, the importance of having a reliable SLN detection methodology to help guide the lymph node sampling process becomes vital.
SLN mapping in lung cancer
While there is ongoing controversy regarding the adequacy of lymph node sampling versus complete lymphadenectomy in lung cancer staging, identification of tumor-associated SLN has also been an area of clinical interest. Some cancers, such as breast (34) and melanoma (35), spread through the lymphatic system and have well-established patterns of spread to SLNs. The concept of SLN biopsy suggests that sampling the first tumor-draining lymph nodes for pathologic analysis can help identify patients who would benefit from adjuvant chemotherapy in addition to limiting the potential morbidity of extensive lymphadenectomy. Studying these patterns of drainage in vivo, especially in patients with diagnosed lung cancer, poses its own set of challenges.
Isosulfan blue dye
Isosulfan blue, which is lipophilic and quickly taken up by the lymphatic system, has been demonstrated to provide reliable identification of SLNs in breast cancer (23) and melanomas (35). This mapping technique was first used for detection of SLN in lung cancer by Little et al. in 1999 (15). Early studies were only able to identify SLN in approximately 50% of patients (Table 2, Figure 1), attributed to the intraoperative learning curve associated with its use, but a high specificity for predicting N0 disease. The difficulty of differentiating between isosulfan blue dye and anthracotic nodes, along with the low success rate in this initial study, has largely precluded further developments in this area.
Table 2
Topol et al. (10)1, % | Riquet et al. (13)1, % | Ndiaye et al. (14)2,3, % | |
---|---|---|---|
Right | |||
Upper | 6.00 | 36.40 | 56.30 |
Apical | 2.40 | 16.70 | 28.10 |
Posterior | – | 10.60 | 9.40 |
Anterior | 3.60 | 9.10 | 21.90 |
Middle | 3.60 | 18.60 | 37.50 |
Lateral | – | 14.00 | 18.80 |
Medial | 3.60 | 4.70 | 16.70 |
Lower | 3.60 | 22.40 | 29.40 |
Superior | – | 6.00 | – |
Medial basal | 3.60 | – | 5.90 |
Anterior basal | – | 1.50 | – |
Lateral basal | – | 1.50 | 5.90 |
Posterior basal | – | 7.50 | 17.70 |
Left | |||
Upper | 7.20 | 33.60 | – |
Apicoposterior | 1.20 | 13.90 | |
Anterior | 2.40 | 7.40 | |
Superior lingular | 1.20 | 6.60 | |
Inferior lingular | 2.40 | 5.70 | |
Lower | 0 | 16.10 | – |
Superior | – | 5.10 | |
Medial basal | – | – | |
Anterior basal | – | 1.70 | |
Lateral basal | – | 3.40 | |
Posterior basal | – | 5.90 |
1, direct drainage pathways/pathways injected; 2, number of segments with direct drainage pathways/segments injected; 3, study only included right lung segments.
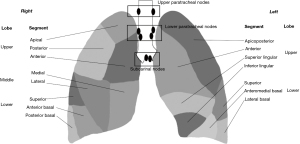
Radioisotope
SLN identification utilizing radioisotopes requires preoperative CT guided injection of a radiocolloid, which occurs between 6–24 h prior to surgery (36,37). In a study by Liptay et al. in 2000 (16), the team utilized a radioisotope for SLN identification with sensitivity of over 80%, more accurate than the isosulfan blue dye (Table 2). In 2009, this group published the first multicenter trial which ultimately demonstrated an identification rate of 61.5% and accuracy of 83.3% for SLN identification (18). Rzyman et al. (17) presented a series of a larger sample size of patients undergoing radiocolloid lymphatic mapping with SLN biopsy. When combined with immunohistochemical staining, this had a sensitivity of 97% and NPV of 93% to identify SLN, approaching 90% and 95% respectively, which is the accepted standard for clinical use in melanoma and breast cancer. Per this study, the mapping procedure added only 5–8 minutes to the operation time. Nomori et al. (19) published a series of 104 patients with clinical stage I NSCLC who underwent major lung resection and mediastinal lymph node dissection. Utilizing Tc-99 tin radiocolloid, this study was able to identify ex vivo a SLN in 81% of patients.
Some of the difficulties associated with this technique were illustrated by the study conducted by Liptay et al. (18), including: technical failure, aerosolization of radioactivity, and the shine-through effect. The shine-through effect, where signal saturation from the primary tumor bleeds into the surrounding area, also can make it difficult to identify smaller foci of nearby signal (37). Other difficulties associated with this technique include dissection which causes extravasation of blood that can contaminate the area being measured for radiocolloid. In addition, when the central tumor is close to the lymph nodes, placement of the probe in proximity to the injection site becomes difficult. The variability of SLN identification has led to more recent studies focusing on techniques which help mitigate some of these aforementioned difficulties while ensuring a more consistent SLN identification rate.
Near infrared imaging (NIR)
NIR is another technique for real-time intraoperative visual mapping utilizing a fluorescent tracer, indocyanine green (ICG). When compared to radioisotope tracers, NIR has lower absorption, scatter, and tissue autofluorescence within the NIR spectrum (22,23,37), which along with reduced radioactivity effect on patients and surgeons (20), makes it ideal for intraoperative imaging. Studies have demonstrated SLN identification rate of 80–100% (21-23) utilizing this technique (Table 2). Yamashita et al. reported an 80.3% SLN identification rate, Gilmore et al. reported a 100% SLN identification rate with an optimized ICG dose, and Moroga et al. reported an 80% SLN identification rate with a false negative of 0% in a retrospective study comparing patients who underwent segmentectomy with and without SLN biopsy utilizing NIR. More recently, Hachey et al. in a series of 12 patients with T1N0 NSCLC, reported an 80% SLN detection rate with the addition of navigational bronchoscopy (NB) for guidance (23). This study utilized a pre-operative chest CT or PET/CT scan to identify the lesion of interest and with NB, guide the injection of ICG to the deep margin of the lesion. Following injection, this ICG “tattoo” was able to be visualized with NIR and removed during lung resection. Overall, these studies demonstrated a consistent success rate with SLN identification. They also reported similar patterns of SLN based on tumor location, though with enough variability as to warrant the need for intraoperative SLN mapping.
A major limitation with utilizing NIR is frequent spillage of ICG during injection, which can increase the background signal, similar to the shine-through effect reported with the radiocolloids. Furthermore, the injection can only be performed once, as this also increases the background signal.
Conclusions
The lymphatic drainage of lung cancer has implications in both the diagnosis and treatment of early stage lung cancer. As we come to better understand the mechanisms driving the spread of tumor through the lymphatic system and the ways in which it interacts with the surrounding environment, we become better equipped to develop technologies that can improve both detection and interventions. As imaging modalities such as PET/CT and EBUS continue to improve, systematic approaches to nodal sampling by EBUS-TBNA, as described by Murthi et al. (31), provide a constructive next step in defining quality outcomes in the use of this staging technology. Another possible extension of intraoperative near-infrared lymphatic mapping includes studying the feasibility of NIR SLN detection using the da Vinci Surgical System (Intuitive Inc., Sunnyvale, CA, USA) which has integrated fluorescence detection via “Firefly” mode, a method which has been studied in cancers of the gastrointestinal tract (38-40).
As presented in a review by Kachala et al. (41), improvements in lymph node imaging naturally leads to greater possibilities in lymph node targeted therapies. Some of these modalities include: herpes virus (42), oncolytic viruses (43), and adoptive T-cell therapy (41). While these methods are being studied largely within pre-clinical models, they provide a taste of the therapeutic possibilities when combining improved detection with targeted therapy.
Ultimately, as we attempt to improve the long-term disease-specific survival after surgical resection for NSCLC, understanding the lymphatic drainage of these tumors can help us develop better targeted diagnostic and therapeutic interventions.
Acknowledgments
Funding: None.
Footnote
Provenance and Peer Review: This article was commissioned by the Guest Editor (Kei Suzuki) for the series “Immune Response in Lung Cancer” published in AME Medical Journal. The article has undergone external peer review.
Peer Review File: Available at https://amj.amegroups.com/article/view/10.21037/amj-20-172/prf
Conflicts of Interest: The author has completed the ICMJE uniform disclosure form (available at https://amj.amegroups.com/article/view/10.21037/amj-20-172/coif). The series “Immune Response in Lung Cancer” was commissioned by the editorial office without any funding or sponsorship. The author has no other conflicts of interest to declare.
Ethical Statement: The author is accountable for all aspects of the work in ensuring that questions related to the accuracy or integrity of any part of the work are appropriately investigated and resolved.
Open Access Statement: This is an Open Access article distributed in accordance with the Creative Commons Attribution-NonCommercial-NoDerivs 4.0 International License (CC BY-NC-ND 4.0), which permits the non-commercial replication and distribution of the article with the strict proviso that no changes or edits are made and the original work is properly cited (including links to both the formal publication through the relevant DOI and the license). See: https://creativecommons.org/licenses/by-nc-nd/4.0/.
References
- Naruke T, Goya T, Tsuchiya R, et al. Prognosis and survival in resected lung carcinoma based on the new international staging system. J Thorac Cardiovasc Surg 1988;96:440-7. [Crossref] [PubMed]
- Bille A, Woo KM, Ahmad U, et al. Incidence of occult pN2 disease following resection and mediastinal lymph node dissection in clinical stage I lung cancer patients. Eur J Cardiothorac Surg 2017;51:674-9. [Crossref] [PubMed]
- Martini N. Mediastinal lymph node dissection for lung cancer. The Memorial experience. Chest Surg Clin N Am 1995;5:189-203. [PubMed]
- Darling GE, Allen MS, Decker PA, et al. Randomized trial of mediastinal lymph node sampling versus complete lymphadenectomy during pulmonary resection in the patient with N0 or N1 (less than hilar) non-small cell carcinoma: results of the American College of Surgery Oncology Group Z0030 Trial. J Thorac Cardiovasc Surg 2011;141:662-70. [Crossref] [PubMed]
- Little AG, Rusch VW, Bonner JA, et al. Patterns of surgical care of lung cancer patients. Ann Thorac Surg 2005;80:2051-6; discussion 2056. [Crossref] [PubMed]
- Suzuki K, Kachala SS, Kadota K, et al. Prognostic immune markers in non-small cell lung cancer. Clin Cancer Res 2011;17:5247-56. [Crossref] [PubMed]
- Hugues S, Fetler L, Bonifaz L, et al. Distinct T cell dynamics in lymph nodes during the induction of tolerance and immunity. Nat Immunol 2004;5:1235-42. [Crossref] [PubMed]
- Cochran AJ, Huang RR, Lee J, et al. Tumour-induced immune modulation of sentinel lymph nodes. Nat Rev Immunol 2006;6:659-70. [Crossref] [PubMed]
- Stump B, Cui Y, Kidambi P, et al. Lymphatic Changes in Respiratory Diseases: More than Just Remodeling of the Lung? Am J Respir Cell Mol Biol 2017;57:272-9. [Crossref] [PubMed]
- Topol M, Masłoń A. The problem of direct lymph drainage of the bronchopulmonary segments into the mediastinal and hilar lymph nodes. Clin Anat 2009;22:509-16. [Crossref] [PubMed]
- Kim AW. Lymph node drainage patterns and micrometastasis in lung cancer. Semin Thorac Cardiovasc Surg 2009;21:298-308. [Crossref] [PubMed]
- Rouvière H. Anatomie des lymphatiques de l’homme. Journal of the American Medical Association. Paris: Masson; 1932:489.
- Riquet M, Hidden G, Debesse B. Direct lymphatic drainage of lung segments to the mediastinal nodes. An anatomic study on 260 adults. J Thorac Cardiovasc Surg 1989;97:623-32. [Crossref] [PubMed]
- Ndiaye A, Di-Marino V, Ba PS, et al. Anatomical variations in lymphatic drainage of the right lung: applications in lung cancer surgery. Surg Radiol Anat 2016;38:1143-51. [Crossref] [PubMed]
- Little AG, DeHoyos A, Kirgan DM, et al. Intraoperative lymphatic mapping for non-small cell lung cancer: the sentinel node technique. J Thorac Cardiovasc Surg 1999;117:220-4. [Crossref] [PubMed]
- Liptay MJ, Masters GA, Winchester DJ, et al. Intraoperative radioisotope sentinel lymph node mapping in non-small cell lung cancer. Ann Thorac Surg 2000;70:384-9; discussion 389-90. [Crossref] [PubMed]
- Rzyman W, Hagen OM, Dziadziuszko R, et al. Intraoperative, radio-guided sentinel lymph node mapping in 110 nonsmall cell lung cancer patients. Ann Thorac Surg 2006;82:237-42. [Crossref] [PubMed]
- Liptay MJ, D'amico TA, Nwogu C, et al. Intraoperative sentinel node mapping with technitium-99 in lung cancer: results of CALGB 140203 multicenter phase II trial. J Thorac Oncol 2009;4:198-202. [Crossref] [PubMed]
- Nomori H. Sentinel node mapping in lung cancer: the Japanese experience. Semin Thorac Cardiovasc Surg 2009;21:316-22. [Crossref] [PubMed]
- Yamashita S, Tokuishi K, Miyawaki M, et al. Sentinel node navigation surgery by thoracoscopic fluorescence imaging system and molecular examination in non-small cell lung cancer. Ann Surg Oncol 2012;19:728-33. [Crossref] [PubMed]
- Moroga T, Yamashita S, Tokuishi K, et al. Thoracoscopic segmentectomy with intraoperative evaluation of sentinel nodes for stage I non-small cell lung cancer. Ann Thorac Cardiovasc Surg 2012;18:89-94. [Crossref] [PubMed]
- Gilmore DM, Khullar OV, Jaklitsch MT, et al. Identification of metastatic nodal disease in a phase 1 dose-escalation trial of intraoperative sentinel lymph node mapping in non-small cell lung cancer using near-infrared imaging. J Thorac Cardiovasc Surg 2013;146:562-70; discussion 569-70. [Crossref] [PubMed]
- Hachey KJ, Digesu CS, Armstrong KW, et al. A novel technique for tumor localization and targeted lymphatic mapping in early-stage lung cancer. J Thorac Cardiovasc Surg 2017;154:1110-8. [Crossref] [PubMed]
- Fourdrain A, Lafitte S, Iquille J, et al. Lymphatic drainage of lung segments in the visceral pleura: a cadaveric study. Surg Radiol Anat 2018;40:15-9. [Crossref] [PubMed]
- Mori M, Andersson CK, Graham GJ, et al. Increased number and altered phenotype of lymphatic vessels in peripheral lung compartments of patients with COPD. Respir Res 2013;14:65. [Crossref] [PubMed]
- Young RP, Hopkins RJ, Christmas T, et al. COPD prevalence is increased in lung cancer, independent of age, sex and smoking history. Eur Respir J 2009;34:380-6. [Crossref] [PubMed]
- Zhong G, Chen L, Yin R, et al. Chemokine (C‑C motif) ligand 21/C‑C chemokine receptor type 7 triggers migration and invasion of human lung cancer cells by epithelial‑mesenchymal transition via the extracellular signal‑regulated kinase signaling pathway. Mol Med Rep 2017;15:4100-8. [Crossref] [PubMed]
- Ding N, Mao Y, Gao S, et al. Predictors of lymph node metastasis and possible selective lymph node dissection in clinical stage IA non-small cell lung cancer. J Thorac Dis 2018;10:4061-8. [Crossref] [PubMed]
- D'Cunha J, Herndon JE 2nd, Herzan DL, et al. Poor correspondence between clinical and pathologic staging in stage 1 non-small cell lung cancer: results from CALGB 9761, a prospective trial. Lung Cancer 2005;48:241-6. [Crossref] [PubMed]
- Lilo MT, Allison DB, Younes BK, et al. The critical role of EBUS-TBNA cytology in the staging of mediastinal lymph nodes in lung cancer patients: A correlation study with positron emission tomography findings. Cancer Cytopathol 2017;125:717-25. [Crossref] [PubMed]
- Murthi M, Donna E, Arias S, et al. Diagnostic Accuracy of Endobronchial Ultrasound-Guided Transbronchial Needle Aspiration (EBUS-TBNA) in Real Life. Front Med (Lausanne) 2020;7:118. [Crossref] [PubMed]
- Zheng H, Xie HK, Li C, et al. Radical mediastinal nodal removal improves disease-free survival for pulmonary low-grade malignant tumors. Lung Cancer 2012;75:342-7. [Crossref] [PubMed]
- Ray MA, Smeltzer MP, Faris NR, et al. Survival After Mediastinal Node Dissection, Systematic Sampling, or Neither for Early Stage NSCLC. J Thorac Oncol 2020;15:1670-81. [Crossref] [PubMed]
- Krag DN, Weaver DL, Alex JC, et al. Surgical resection and radiolocalization of the sentinel lymph node in breast cancer using a gamma probe. Surg Oncol 1993;2:335-9; discussion 340. [Crossref] [PubMed]
- Morton DL, Wen DR, Wong JH, et al. Technical details of intraoperative lymphatic mapping for early stage melanoma. Arch Surg 1992;127:392-9. [Crossref] [PubMed]
- Soltesz EG, Kim S, Laurence RG, et al. Intraoperative sentinel lymph node mapping of the lung using near-infrared fluorescent quantum dots. Ann Thorac Surg 2005;79:269-77; discussion 269-77. [Crossref] [PubMed]
- Hachey KJ, Colson YL. Current innovations in sentinel lymph node mapping for the staging and treatment of resectable lung cancer. Semin Thorac Cardiovasc Surg 2014;26:201-9. [Crossref] [PubMed]
- Kobiela J, Bertani E, Petz W, et al. Double indocyanine green technique of robotic right colectomy: Introduction of a new technique. J Minim Access Surg 2019;15:357-9. [Crossref] [PubMed]
- Desiderio J, Trastulli S, Gemini A, et al. Fluorescence image-guided lymphadenectomy using indocyanine green and near infrared technology in robotic gastrectomy. Chin J Cancer Res 2018;30:568-70. [Crossref] [PubMed]
- Spinoglio G, Petz W, Borin S, et al. Robotic right colectomy with complete mesocolic excision and indocyanine green guidance. Minerva Chir 2019;74:165-9. [Crossref] [PubMed]
- Kachala SS, Servais EL, Park BJ, et al. Therapeutic sentinel lymph node imaging. Semin Thorac Cardiovasc Surg 2009;21:327-38. [Crossref] [PubMed]
- Brader P, Kelly K, Gang S, et al. Imaging of lymph node micrometastases using an oncolytic herpes virus and [F]FEAU PET. PLoS One 2009;4:e4789. [Crossref] [PubMed]
- Kishimoto H, Kojima T, Watanabe Y, et al. In vivo imaging of lymph node metastasis with telomerase-specific replication-selective adenovirus. Nat Med 2006;12:1213-9. [Crossref] [PubMed]
Cite this article as: Lin B. Review of patterns of lymphatic drainage in early-stage non-small cell lung cancer. AME Med J 2021;6:41.